Nuclear Zeitgeist Part 1: A Reactor Primer
AI takes a lot of power. Why did we stop it again? Today's deeper dive on Nuclear power and why I think it will power AI in the future.
Nuclear energy is having a moment. Last month, the DOE published a paper about the commercial liftoff of Advanced Nuclear. Larry Ellison mentioned that SMRs (Small modular reactors) will power data centers in the future, and Microsoft will unshutter 3 Mile Island for one last puff of a half-smoked cigarette. Just a few years ago, it was closed for economic reasons.
Interest in nuclear power is rising, and I’m also pretty interested. This is all driven by AI’s insatiable energy demand and the challenging ways to supply power. This is going to be the first in a series about Nuclear Power. Today, I’ll talk about Nuclear Reactors and the basics. In the next post, I’ll write about SMRs and nuclear fuel.
Nuclear energy is a revolution that has been coming for a long time. Atomic energy had a real moment in the 1970s, driven partially by higher gas prices and the maturity of the technology. That came to a grinding halt in the late 1980s after the Chernobyl disaster in 1986, the near misses of Three Mile Island, and the eventual decommissioning of reactors after the Fukushima Daiichi disaster in 2011.
One of the more significant issues and unsung causes was economics; candidly, the entire nuclear industry was in a bit of a bubble in the 1970s. How we solve those issues will determine the industry's future, and that is part of the following reports.
Newer reactor designs were supposed to replace the older designs that caused the problems at the Fukushima GE Light Water Boiling Water-type reactors. A new generation of nuclear reactors was supposed to be safer, more power efficient, and replace older-style boiling water or pressurized water reactors. That new Nuclear reactor was the AP1000, and instead of bringing in a new age of Nuclear, it bankrupted its oldest player, Westinghouse.
The Rise and Fall of Westinghouse
I’ll admit, I didn’t expect to do a deeper dive into Westinghouse as a Nuclear energy primer, but the more I read about it, the more I thought telling the story of Westinghouse was the right way to frame the entire Nuclear energy story because the story of Westinghouse is the story of Nuclear generation in America.
Also, before I begin, this history of the Westinghouse PWR is pretty much definitive. Thank you, Carlos Barrientos. If anyone can get in touch (I tried!), I would love to chat.
I will summarize Carlos' history section heavily because this is all the answers you need. So please don’t pretend that much of this stuff is original; this guy did it first and did it well. I’ll pick up where he left off and link his work repeatedly.
In the beginning, the United States Atomic Energy Commission (AEC) gave the US government a monopoly on all nuclear activity in the United States. After WW2, there were zero nukes, a hugely disruptive technology, and a clear technology tree to progress. That’s exactly what the AEC set out to do.
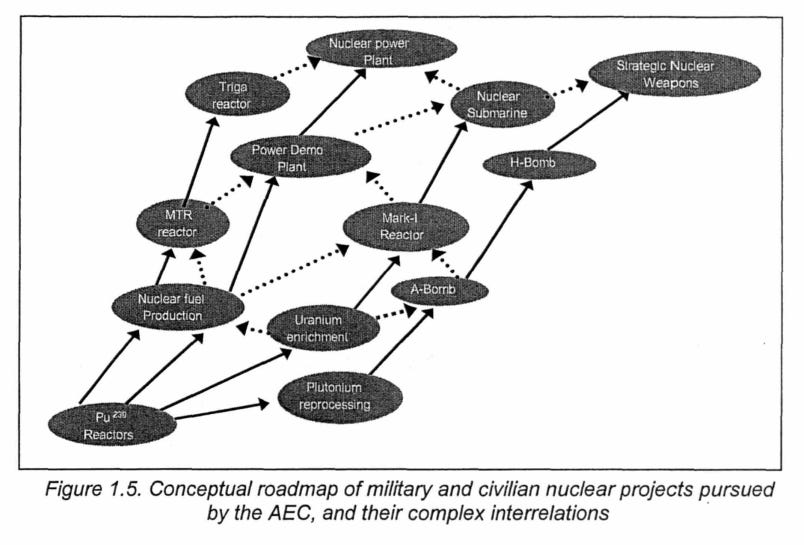
A civilian technology tree (dotted lines) and a military technology tree exist. Still, besides bombs (which will not be discussed), the history of nuclear reactors begins with Mark 1.
Mark 1 and Submarines
Admiral Hyman Rickover developed the S1W / Mark 1 platform, the first pressurized water reactor (PWR). It reached criticality in 1953, and Westinghouse, the contractor of the Mark 1, quickly made the first PWR for commercial use in 1957.
In many ways, this PWR reactor design is history's primary and most successful design. In 1989, it was the vast majority of designs; today, that still holds.
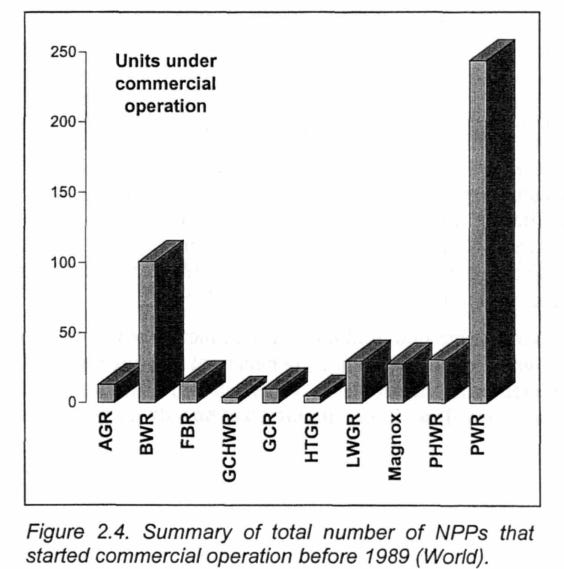
Even today, PWR still accounts for most reactor types and styles. Westinghouse made this possible by licensing its design to multiple players, including B&W and Combustion Engineering. By doing so, it helped make its ecosystem vibrant with numerous suppliers. B&W would become one of the primary suppliers for the Westinghouse ecosystem.
So, let’s talk about PWR because we must understand the past's gold standard to understand the future. I will also briefly discuss BWR and other designs.
Westinghouse’s PWR - Pressurized Water Reactor
The PWR is the grandaddy design of Nuclear reactors. The pressurized water reactor is a two-loop reactor. The primary reactor uses light water as a moderator (to slow neutrons so chain reactions can be sustained) and as a coolant. The fuel is uranium dioxide in zircaloy clad enriched 3.0% U235.
Uranium oxide is a processed form of uranium with more fissile uranium isotype (U235) fuel. The fuel rods are clad in Zircaloyrods filled with pellets of this fuel. Once the critical reaction starts, electrons sustain a slow, sustaining fission process. This sustaining chain reaction creates massive amounts of heat, and the fuel rods must be replaced every 12 to 24 months.
The primary loop uses pressurized light water under 160 bar of pressure. Because of the pressure, it’s never allowed to become steam. This is circulated into a typical steam generator's second loop, often broken into multiple loops to better harvest the power.
The original design was this “simple,” with a pressurizer keeping the water at 160 bar, which creates a closed loop and very little excess radiation. The second loop is just a steam turbine, and steam doesn’t carry much radiation. This was one of the reasons for its popularity; there was little contamination outside the primary loop. PWR uses reliable water as both a moderator and coolant, and it relies on the convenience of pressurized water at 160 bar, which won’t steam! Pretty nifty!
PWR is Westinghouse’s baby. This original design was modified from the Mark 1, and most other PWR designs are derivative designs of Westinghouse. Westinghouse decided on the intake loop out loop heat, the pressure, and the loop pressure. Most PWRs that are not Westinghouse’s are derivatives.
Westinghouse won the day with this design. Westinghouse licensed firms developing the PWR to create an ecosystem of manufacturers so that companies such as Babcock and Wilcox, Mitsubishi, and Combustion Engineering could all make derivatives. This helped develop suppliers and economies of scale so that multiple companies could manufacture the reactor, the NHSS (Nuclear Heat Supply System), and the turbines.
There was no one critical supplier, and utilities could start a PWR with multiple vendors that could step in if another didn’t deliver. This meant each piece could work on its own, and no one company would have to make the entire stack. This makes a lot of sense but would create problems later. In some sense, Westinghouse was the fabless design company, and everyone else was the “fab.” Let’s compare that to GE.
BWR Design - GE Design
GE had the opposite economic setup. They were the sole supplier of their own BWR design. In many ways, they were the IDM (Integrated Device Manufacturer) of the time, and I’m sure they had significant margins from pursuing the vertical strategy. GE was derived from the EBWR reactor from Argonne National Laboratory in 1956, and the prototype was built in 1957. The first commercial nuclear power plant, Dresden 1, was a BWR/1.
The Boiling Water Reactor also used Light water as the modulator and coolant, but instead of creating a secondary loop, the steam would go to the turbine and be brought back into the loop. Condensed cooling water then becomes either water vapor in the cooling tower.
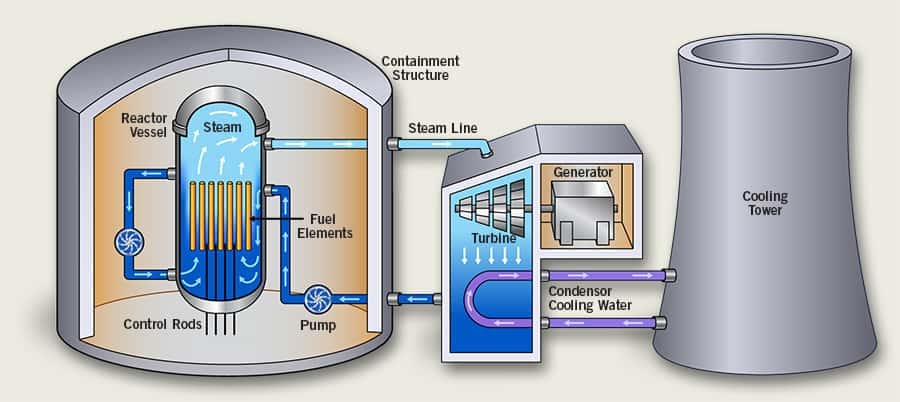
This is a single-loop reactor that brings some of the water outside of the primary containment structure. One downside, however, is that the water that leaves the structure is radioactive, and if the primary loop were to break, this would create an issue. The steam is only reactive for a short time, and multiple layers of protection prevent this from happening.
This single loop is much simpler, but the radioactive water creates complications. The BWR has been improved multiple generations, making the design safer. It has higher power density and better valve controls, improved emergency core cooling systems, and, more recently, some natural circulation for passive safety.
BWR and PWR are the most common reactor designs today. Both have a thermal efficiency in the mid-35 percentage range. Both started as much smaller reactors and then scaled to the 1000 MWH+ range as the designs improved.
Now, the PWR/BWR isn’t the only design in the game, but it is the design for a reason. Both use light water and are simple. But that’s not exactly the only type of reactor out there. Below is a diagram of the moderator, coolant, and fuel types.
Let’s now discuss some of the other designs. They are water-cooled or gas-cooled; the fuel type, coolant, and modulator can also differ.
The most significant difference between most types of reactors is heavy water, light water, or graphite as the moderator. Moderators are substances that slow neutrons to energy levels preferential for fission to prevent neutron absorption so the fission reaction can be sustained. The coolant is the material that takes the heat away from the reactor and is often converted into energy via a steam turbine.
Specific designs allow for natural Uranium as a fuel type, such as the Magnox design, the popular PHWR, or the Pressurized Heavy Water Reactor. PHWR uses D2O (deuterium oxide) or heavy water. It has a higher density and melting point and is an adequate coolant and modulator of nuclear reactions. That said, it’s harder to use than good old light water. These reactors power some of Canada's and India’s nuclear reactors. They can be fueled by natural uranium, so no refinement is needed. However, heavy water is costly.
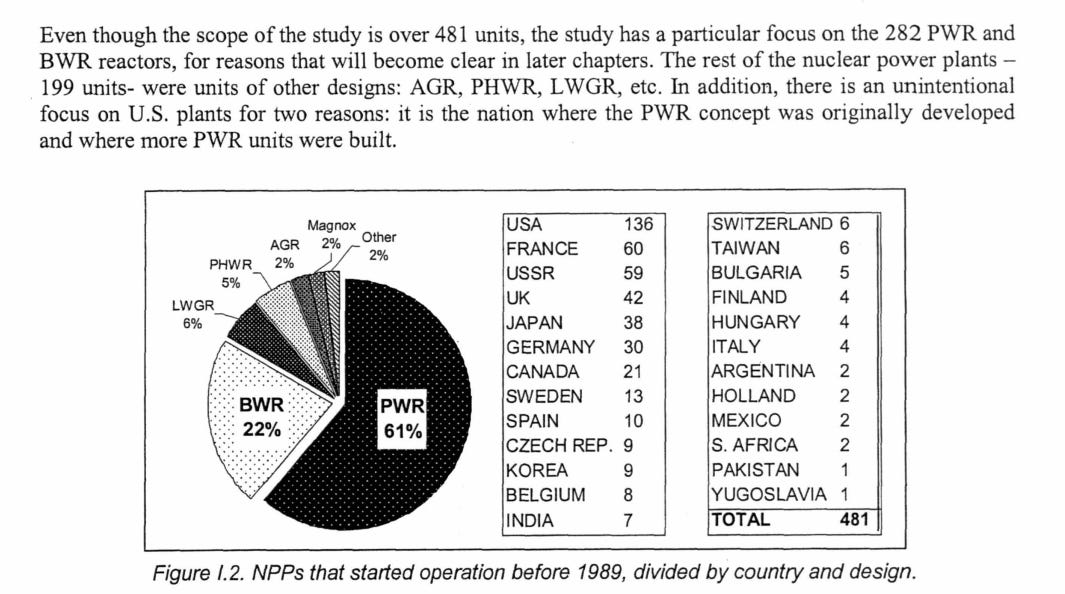
There’s also the LWHR, or light water graphite moderated reactor. This is one of the rarer designs and was famously responsible for the Chernobyl reactor meltdown. It was cheap and had design flaws in the void coefficient, yet the USSR built them anyway. It’s less safe than the PWR and BWR designs. That’s the basic overview of all the previous reactor types. Westinghouse won the day, driven by the simple design with a lack of radiation in the second loop, a broader base of suppliers, and a slightly more efficient design. But if the Westinghouse PWR was so good, why did Nuclear halt in the late 1970s?
The Fall of Nuclear (and Westinghouse)
In 1975, nuclear energy looked like the next apparent big thing. Multiple large reactors were coming online and proliferating, and soon, nuclear energy would be America's second largest fuel source after coal.
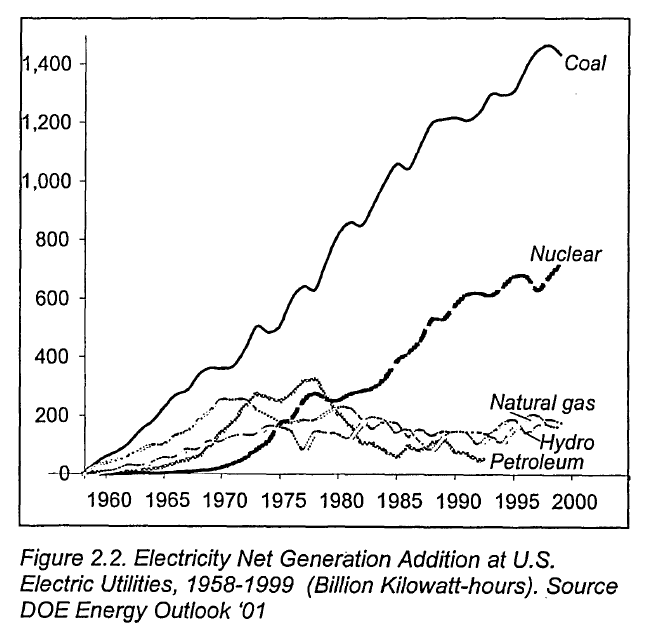
But little did Americans know then that it was the peak. In 1974, 12 reactors came online. Another peak of reactors came online in the mid-80s, but nuclear power started to slow meaningfully after Chernobyl and other incidents weighed heavy on the public’s mind.
However, as noted, nuclear power slowed in the 1970s before these significant events. Why?
The Failure of the AP1000
Before we get into the nitty-gritty details about the AP1000's failure (Nukegate), let’s discuss the negative attributes of a Nuclear power plant. In the 1970s, the time to build a nuclear power plant in the United States started skyrocketing. Below are “overnight costs," which are the total cost assuming the atomic plant would be finished overnight. Costs rose throughout the 1970s.
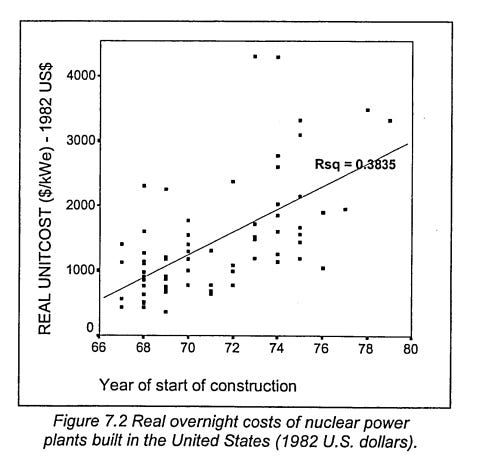
What’s more, construction time was lengthened. Powerplants were becoming more prominent and more powerful, and multiple innovations were added to the design, such as improved safety and simplicity. But this came at the cost of increasing time to market, and this is the critical failure of Nuclear.
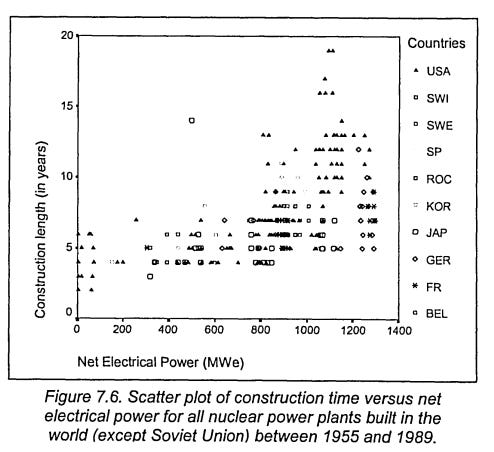
Because Nuclear is almost all capital costs, this chart below breaks it out. If the overnight expenses increase, almost the entire project cost goes up, and it’s all recognized upfront. Nuclear reactors are a fantastic technology, but the more I look at them, the more I realize it’s a financing issue.
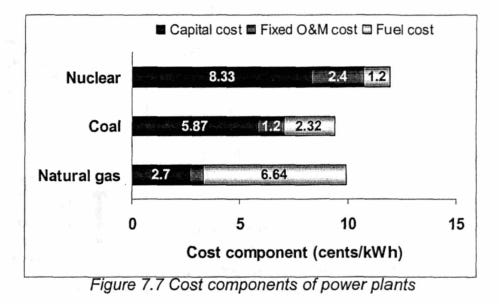
Remember that in the 1970s, runaway inflation and high interest rates were the economic backdrop, and if you had to finance a multiple billion-dollar investment in the 1970s, good luck, my friend. Economics was one of the biggest limiters of Nuclear power, not the technology alone.
But let’s look closer to understand where considerable costs come from. The most significant cost overruns are simple costs, construction materials and equipment, and craft labor. Building the actual plant, not the turbine and NHSS, is costly, and this tends to increase the cost.
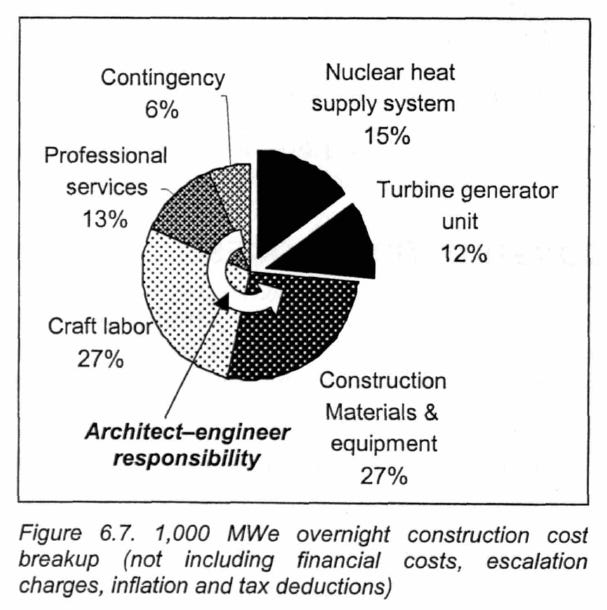
And guess what? The AP1000 / Vogtle plant was the ultimate display of this issue. The AP1000 construction at Vogtle started before the design was finished, and the original aim was to be less expensive than other generation III reactor designs by using less equipment such as valves, pumps, wires, and less land. Additionally, the AP1000 added a passive cooling system, which means it’s the safest.
In any case, the AP1000 was the turbo PWR reactor, the peak of the technology with clever passive cooling and vast economies of scale. Overnight, prices doubled from the original $14 billion to a staggering $35 billion for the two reactors.
Westinghouse was purchased by Toshiba in 2006, which slowed down the project. The AP1000, Westinghouse's crowning achievement and state-of-the-art reactor design, forced the subsidiary into bankruptcy in 2017. The design is still thriving with the CAP1000, a Chinese adaptation of the original AP1000.
But the same things that slowed down nuclear power plants in the 1970s applied to Vogtle 3/4. Overnight capital costs and delays ballooned, raising the total costs. Some of these costs are starting to look like they would be First Of A Kind (FOAK) and should scale over time.
For example, there was a meaningful improvement from Vogtle 3 to Vogtle 4. This means future AP1000s in America could be at a total cost of ~7.5 billion
The DOE believes that recent cost reductions from IRA and the DOE’s LPO programs can lower costs to around ~$96 a MWh, assuming no economies of scale or improvement of cycle times. That’s pretty competitive with energy coming online, and importantly, it’s much more firm than renewable energy.
LCOE stands for levelized cost of energy, and in the case of Nuclear energy, this would be over its capital cost period. However, LCOE often fails to capture the total energy cost for Nuclear power plants, which have 80-year lives. The problem remains. Nuclear energy is an excellent option for clean power generation but is a hefty check.
Even with the cost reductions, nuclear power isn’t precisely cost-competitive with solar or wind.
But again, it's consistent, which Wind and Solar are not. Nuclear is a match made in heaven for AI. 100% uptime for significant training and inferencing is the perfect use case. Remember that in recent PJM auctions, the price for delivery throughout the region soared to $269 MW-day, up from $28.92 MW-day the year before. A 10x creates a price umbrella for Nuclear to thrive.
AI is the real game changer. If you’re going to be putting down a million GPU clusters, power availability and density are going to be huge. Multiple hyperscalers are planning for more prominent than 1 GW clusters, and the current power generation in the US has been flat for almost a decade.
AI is a critical new power generation source that can’t use renewable power without massive battery storage. AI is the most significant new incremental power demand; it requires firm control, and it would be ideal not to boil the ocean to generate tokens. Every hyperscaler has expressed interest, and now is the time for Nuclear power.
In the following reports of "Nuclear Zeitgeist," I’ll discuss SMRs, why nuclear is now, and different fuel types. Before diving deeper into the market, I wanted to provide a free reactor-type primer. You’ll have to understand PWR before you can understand SMRs.
You’ll be hearing more about this topic very soon.
Sources
DOE Pathways to Commerical Liftoff: Advanced Nuclear
Westinghouse PWR: The rise and fall of a dominant design in the electric power industry
Boiling Water Reactor Technology - International Status and UK Experience
Eh. I covered the issue for ten years and went from pro-nuclear to nuclear skeptic. You hit a big part of it on the head: economically, nuclear can't compete with renewables except in powerpoint presentations. But there is also the long history of design mishaps. Even what should be no brainer things like water containment pools got messed up at Vogtle. (Ask NuScale about their passive cooling system.) 60%+ pct of the costs are steel, cement and engineering: good luck getting a Moore's Law for that! Nuclear plays on people's nostalgia for Pax Americana. Solar and storage will be cheaper and you won't have to wait 15 years to use it. Put it another way: every AI technology we know today will be gone and obsolete before an AI nuke comes up.
I am wondering when to updated